Coll Antropologorum. 2015;39(3):667-73 (CC)
1Mechanical Engineering Faculty in SlavonskiBrod, University of Osijek, Osijek, Croatia, EU 2Klapan Medical Group University Polyclinic, Zagreb, Croatia, EU, and the School of Medicine University of Osijek, Republic of Croatia, EU
ABSTRACT
In the paper we are introducing guidelines and suggestions for use of 3D image processing SW in head pathology diagnostic and procedures for obtaining physical medical model by additive manufacturing/rapid prototyping techniques, bearing in mind the improvement of surgery performance, its maximum security and faster postoperative recovery of patients. This approach has been verified in two case reports. In the treatment we used intelligent classifier-schemes for abnormal patterns using computer-based system for 3D-virtual and endoscopic assistance in rhinology, with appropriate visualization of anatomy and pathology within the nose, paranasal sinuses, and scull base area.
Key words: virtual reality, virtual endoscopy, additive manufacturing, rapid prototyping, medical models, medical imaging, 3D imaging, rhinology, paranasal sinuses, engineering
Introduction
Extremely valuable information on anatomic relationships in the nose, paranasal sinuses and scull base, while planning and performing endoscopic, and/ortele-endoscopic surgery, is provided by high quality MSCT (MultisliceComputedTomography) or MRI (Magnetic Resonance Imaging) diagnosis1, thus contributing greatly to the safety of this kind of surgery2.
The diagnosis should include precise multi slice MSCT scanning in axial and coronal sections to get an approximate insight into the main characteristics of the anatomical relationship between vital structures of the head (i.e. skull base, carotid artery, optic nerve). Strictly following these criteria, tissues of different density at these anatomical locations can be differentiated. Neither standard roentgenograms nor lateral tomograms or even MSCT slices of the highest quality of the region can provide a comprehensive insight into the local finding3.
Once created from 2D cross-section images with 3D modelling software, virtual 3D surface models or volume rendered models can be further used in virtual reality(VR).Our final experience in the field of use appropriate virtual medical models, indicate that the entire diagnostic and/or operative procedure can be completely simulated, and critical areas avoided during the real procedure by employing real patient images in the operation preparatory phase using complex spatial models and simulated operative field entry (RP/ rapid prototyping models, VE/virtual endoscopy, VS/virtual surgery)4.
During our diagnostic and/or surgical activities of different cases, we used intelligent classifier-schemes for abnormal patterns using computer-based system for 3D-virtual and endoscopic assistance in rhinology,with appropriate visualization of anatomy and pathology within the nose, paranasal sinuses, and scull base area.Software (SW), used in mentioned activities, based on strictly defined algorithm, once when tested and approved will be nominated for use in regular rhinologic application, with explanation of all benefits in this new process.
Medical Models
In human medicine, extremely valuable information on anatomic relationships in particular regions while planning and performing endoscopic surgery is provided by high quality CT or MRI diagnosis. Once created from 2D-CT/MRI cross-section images with 3D modelling SW, virtual 3D surface models or VR models can be further used in VR for measuring, operation planning, simulations and structural finite element analysis (FEA) (Figure 1).
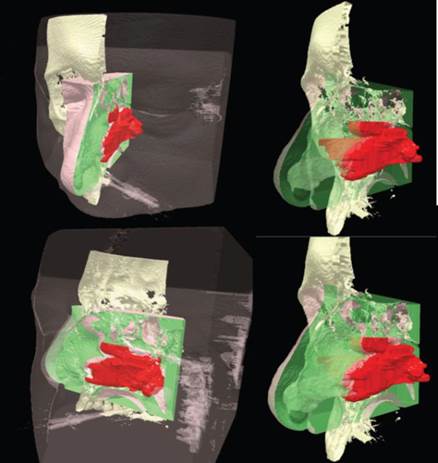
However, virtual 3D models also can be used in actual reality for tangible, physical (real) models obtained e.g.by rapid prototyping (RP) technique. RP techniques look most promising to satisfy medical need for tangible models. While prototyping is a usually slow and expensive process of building pre-production models of a product to test various aspects of its design, RP techniques are methods that allow quick production of physical prototypes (Figure 2).
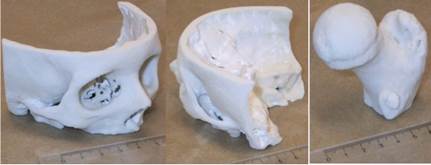
Nowadays even more often, RP techniques provide to medicine actual products e.g. prosthesis and implants with the important benefit in significant shortening of the Time to Market5,6.The mode of computer visualization of anatomic structures7 of the human body used to date could only provide diagnostic information and possibly assist in the preoperative preparation.
Additive manufacturing technology The comparably young manufacturing technology of layer-based automated fabrication process for making 3D physical objects directly from 3D CAD data set was originally called Rapid Prototyping (RP) when the first commercial process – “stereolitography”was entered the market in 1987.This technology is still frequently called “rapid prototyping”,but in the past years many different names have been introduced for these new and innovative manufacturing technologies, including: Desktop Manufacturing, On-Demand Manufacturing, Freeform Manufacturing, Digital Fabrication, Layer Manufacturing, Direct Manufacturing and many others. Today, the term Additive Manufacturing (AM) for these advanced technologies seems to be the most accepted one8.
An important difference between Additive Manufacturing and two other traditional production techniques – Subtractive Manufacturing (milling, turning etc.) and Formative Manufacturing (casting, forging etc.) is the fact that all of these new techniques build parts by adding material layer by layer. Today, there are many different additive manufacturing techniques with high accuracy and large choices of materials available on the market. However, some of specially developed AM processes are still not commercialized. The most successfully developed techniques are: Stereolithography (SLA), Selective Laser Sintering (SLS), Laminated Object Manufacturing (LOM), Fused Deposition Modelling (FDM), 3D Printing etc.5.
In an additive manufacturing process, the product is firstly designed on a computer and then created based on the computer data. Therefore an essential prerequisite is the digital computer representation, usually made in a 3D geometrical modelling computer system like a CAD system, a 3D scanner, computed tomography, etc. Precision of a computer representation is a key parameter for controlling the tolerances of the future model.
During last two decades techniques of additive manufacturing have been tested and used in many different areas in medicine9,10. Such areas include:
• The physical models of human organs those are extremely effective in realizing the anatomy and enhancing discussion and collaboration among teachers, students, researchers and physicians;
• Virtual planning and simulation of operation for orthopaedic surgery, vascular surgery and maxilla surgery with complex spatial relationships;
• Prosthesis like titanium dental cast crowns, free of porosity, with excellent functional contour and a smooth surface finish, could be obtained from the first casting trial;
• Customized implants are very promising and more researched field of AM application. There are many already adopted applications, such as titanium human skull implants and hearing aid shells;
• Biomedical devices like a rapid produced polymeric implant consisted of a drug embedded in a polymeric matrix that was surgically implanted into the body.
Some of common advantages of additive manufacturing techniques when used in medicine are: speed; manufacturing flexibility; high degree of control over part microstructure; wide variety of engineering and medical materials.
Existing additive manufacturing processes also have some common shortcomings like: lack of required mechanical properties depending on material combination; low accuracy; high computational demands and poor bio-compatibility. Yet, most of listed shortcomings can be successfully avoided in particular case with proper selection of techniques and materials11-13.
Additive manufacturing of physical medical models Basically, process chain of layer-based additive manufacturing is illustrated in Figure 3. Process starts with a computer (virtual) 3D data set representing the object to be produced. A complete and error-free 3D data set (also called virtual object model) is absolutely prerequisite for successful additive manufacturing of physical products.In engineering, the data set is mostly obtained by designing of object in professional 3D CAD systems (e.g. Autodesk Inventor, ProEngineer, Catia etc.). However, the data set could be also obtained by the Reverse Engineering (RE) technique.
Independently of how it was obtained, the 3D data set is first sliced into layers, using a specializedSW. As a result, a set of contoured virtual slices of even thickness is obtained (Figure 3).
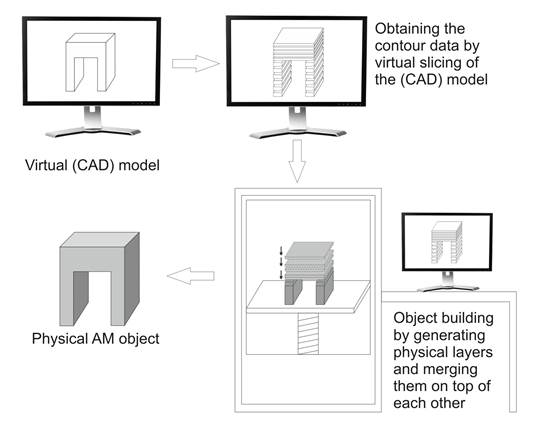
The data set, consisting of the contour data (x-y plane), the layer thickness (z axis) and the layer number of each layer is submitted to a machine that executes two elementary process steps per layer in order to create a part. First, each layer is processed according to the given contour and layer thickness data (Figure 3). This can be done in many ways using different physical principles depending of what AM technique has been using. In the second step, each layer is bonded to the preceding layer, now forming the top layer of the partly finished model. In such way, layer by layer, the physical model is growing from the bottom to the top until the final part is obtained (Figure 3). These basic steps are the same for all of the more than hundred different AM machines available today. The machines differ only by the way each layer is processed and by the way adjacent layers are joined to form the part8.
For medical models, 3D data needed to be obtained in two steps. First, a set of 2D data is acquiring by sophisticated medical imaging technique, such as MSCT, ultrasonography (US), magnetic resonance imaging (MRI, NMRI, MRT) etc. High quality diagnostic image is the main prerequisite for applying AM technique in obtaining medical models. A common format for medical images is DICOM (Digital Imaging and Communication in Medicine). It allows data exchange between multiple medical diagnostic devices as well as between diagnostic devices and computer networks. Before the introduction of DICOM standards, image recordings were stored on films, where the information obtained from the diagnostic device was in part lost. In the second step, special SW (e.g. 3D-Doctor, Mimics) is then using to create accurate virtual 3D CAD model of anatomical structure from the set of 2D medical imaging data(Figure 4).
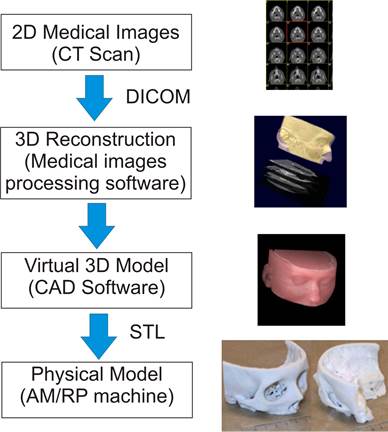
The format of created 3D data set of a virtual model has to be compatible with AM machine software. Admissible data formats include native formats of various CAD systems, some other 3D modelling formats (e.g. VRML) and so-called STL format. STL (Standard Tessellation Language or STereoLithography) format is nowadays regarded practically as an industry standard. It can be exported from almost any 3D CAD systems and from almost any scanner and also imported and processed by any available AM machine (Fig. 4).In STL data set every surface (inner and outer) of the virtual model is approximated by triangles of different sizes aiming to reach an optimum fit. STL is very simple data format in which every triangle is defined by just four data (three node points and one normal vector defining the orientation of the surface (inner/outer). Because of the triangles, the data set can simply be sliced in any desired coordinate direction in order to receive the contours of each layer. The STL data are either exported by the CAD system/Medical images processing software or are calculated by rapid prototyping software8.
Next, appropriate AM material and additive manufacturing technique have to be selected. Because of the good surface quality and the detailed reproduction, AM techniques based on generating a solid layers by polymerization, such as stereolithography and polymer jetting/printing are used preferably to make physical medical models of sculls and other human bone structures. Interior hollow structures such as frontal sinus or the filigree sub-cranial bone structures can be facsimiled best by these AM techniques. However, laser sintering, fused layer manufacturing, layer laminate manufacturing or 3D printing techniques deliver medical models as well and are frequently used for this purpose. For sintering and fused layer manufacturing (e.g. FDM) methods there are special, approved materials for medical use available that can be sterilized8.
3D printing technique delivers slightly less details and a rougher surface comparing to stereolitography. The models obtained by 3D printing are not transparent too (Figure 2), but this AM technique is fast and cheap (Figure 5).
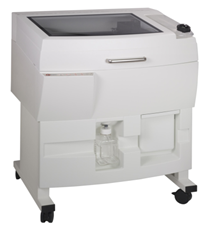
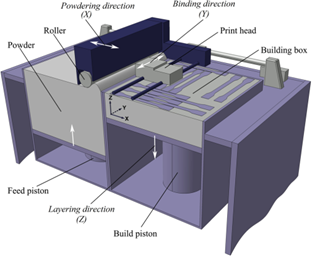
The decision which AM technology to use depends mainly on whether the part must be transparent, what surface quality is requested, whether it needs to be sterilized, how to address sustainability, do medical doctors need models for training/surgery planning or implanting into a patient, and of course, how big is the budget?
Methods and Equipment MSCT Scanning Sinus MSCT scan in coronal projection is a term familiar to every radiologist dealing with MSCT in the world, and the layer thickness, shift, gantry, and window are internationally standardized and accepted values, thus being reproducible all over the world14.But on the other side, the basic MSCT diagnostics has also limited possibilities, first of all because it presents summation images in a single plane (layer) but cannot present the continuity of structures15. The intraoperative use of computer in real time requires development of appropriate hardware (HD) and SW to connect medical instrumentation with the computer, and to operate the computer by thus connected instrumentation and sophisticated multimedia interfaces. In rhinology, such a revolutionary approach is of paramount importance for the surgeon because of the proximity of intracranial structures and limited operative field layout hampering spatial orientation during the “standard” operative procedure4,14.
The complex SW systems allow visualization and/or tele-visualization of MSCT or MRI section in its natural course (the course of examination performed), or in an imaginary, arbitrary course. Particular images can be transferred, processed and deleted, or can be presented in animated images, as already shown16.
Other equipment For medical imaging in reported cases we have been used a computer workstation HP Z600 Workstation with dual Intel Xeon microprocessors at 2.4 GHz; 16 GB system memory and NVIDIA Quadro 600 professional graphic adapter and installed operating system Microsoft Windows 7 Enterprise 64-bit.Medical imaging software used in reported cases were 3D-DOCTOR. It is an advanced 3D modeling, image processing, and measurement software for MRI, CT, PET, microscopy, and industrial 3D imaging applications17.Sample images were analyzed in 3 dimensions in selected medical imaging SW.
Case Reports A 29-year-old female with chronic sinusitis was examined as the first case. MSCT of the sinuses in coronal, sagital and axial sections showed disease of the ethmoidal infundibulum dextrolateraly with homogenous opacification in the region of the right anterior ethmoids and the nasal cavity, epipharynx and partialy in oropharyngeal region (dg.Antrochoanal polyp).A huge middle turbinate was noticed on the right.The right maxillary sinus with no evidence of the disease.Retention in the region of the anterior and posterior ethmoidal cells, with frontal and sphenoidal sinuses and the left maxillary sinus of normal transparency.Intact bony borders of the orbits.Patient also complained of difficulty breathing easily, with additional headaches in the right fronto-ethmoidal region, and recurrent sinusitis with postnasal dripping (appropriate nasal discharge/rhinosecretion).
During the diagnostic process, we used the standard 2D-MSCT imaging sections, virtual endoscopy of the patient’s head model over a few applied travel sections through patient's “virtual” head, physical RP models of patient’s head showing a clear demarcation between the diseased and healthy tissues in the projection of the nose, paranasal sinuses and skull base (Figure 6).
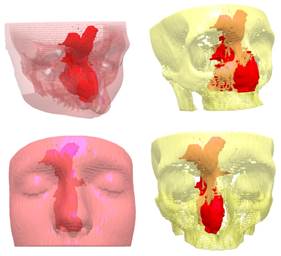
During surgery, we profited byall advanced tools mentioned before, describing the expansion of the pathological process, and we were therefore able to timely, valid and reliably conclude that we have done the procedure correctly, within the limits of surgical normal tissue, without the additional trauma of surrounding tissue of anatomical region which has undergone surgical treatment. Thus we have, among other things, enable and significantly shorter postoperative hospital stay of patients (several hours), and significantly faster recovery after surgery (3 days) (Figure 7).
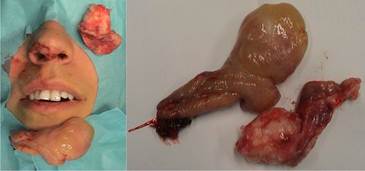
A 53-year-old male with chronic left maxillary sinusitis was examined as the second case. MSCT of the sinuses in coronal, sagital and axial sections showed disease of the left maxillary sinus, with homogenous opacificationof all anatomical space of the sinus (dg. Aspergilloma of the left maxillary sinus, known also as a mycetoma or fungus ball). OMC bilaterally, with no suspected spread of the disease to the frontal or sphenoetmoidalrecess. A huge middle turbinate was noticed on the left. A minor retention cyst observed in the region of the alveolar recess of the right maxillary sinus, with no signs of inflammatory changes. Frontal and sphenoidal sinuses, as well as anterior and posterior ethmoidal cells of normal transparency.Intact bony borders of the orbits. The patient reported facial headaches in the left maxillary sinus region, and recurrent sinusitis with postnasal dripping.
During the diagnostic process, we used the same approach as in the treatment of the first patient. During surgery, we had a very clear view of the size, shape and anatomical characteristics of pathological process and the status of the sinus ostium and sinus periosteum involvement in the maxillary sinus cavity (Figure 8).
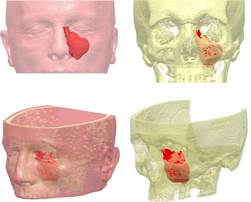
In this way we could very easily be able to apply the minimum invasive traumatic approach to the sinus cavity, keeping in mind the safety of patients and the feasibility of the operation. Thus we have enabled, as in the case of the first patient described, significantly shorter postoperative hospital stay of patient (several hours), and his rapid recovery after surgery (3 days).
Discussion Use of the latest program systems enables development of 3D spatial models, exploration in various projections, simultaneous presentation of multiple model sections and, most important, model development according to open computer standards18. Such a preoperative preparation can be applied in a variety of program systems that can be transmitted to distant collaborating radiologic and surgical work sites for preoperative consultation as well as during the operative procedure in real time (telesurgery, tele-FESS)4. During our activities we used a new lossless image compression algorithm which is based on highly adaptive prediction and contextual error modelling, which allows modelling of more complex image structures such as nontrivially oriented edges and the periodicity and coarseness of textures, and the main advantage of applied method is the increase in lossless compression ratio of image data16,19. Results obtained with proposed compression method on medical images are very encouraging, beating several well-known and used lossless compression methods, which indicates that the proposed predictor can also be used in other image processing applications such as segmentation and extraction of image regions19.
Intended use of new technologies in the diagnostic and surgical rhinology processes, as noted in our two clinical cases described, allows not only the planning of operational process, but also precisely define or choose the type of surgery (e.g. minimally invasive endoscopic 3D-computerized navigation surgery ). Of course, everything must be coordinated and agreed with a number of important factors that strongly determine the choice of surgical treatment, such as the anatomical location of the pathological process/cancer sites, extent of pathological processes and involvement of normal structures (mucosa, bone, cartilage, blood vessels, nerves and etc.). In this way we have allowed the freedom to modify and / or modify the form of surgical intervention, and thus the possibility of opening / exploration of the whole or only part of the anatomical regions where a pathological substrate is. This ultimately means that we have the possibility of modifying criteria of certain surgical procedures or interventions that have so far been medically clearly defined and determinate, without prejudice to the safety and/or successful performance of the surgery. All this provides a significant reduction in "surgical trauma" of patients, reduces recovery time after surgery, such as accelerating the recovery time of patients.
Conclusions As presented in two case reports, systematic approach combined with modern tools for medical imaging and additive manufacturing provides: Ø Computer-based system for virtual endoscopic assistance in rhinology Ø Extending physician’s visualization and orientation in the anatomical space of the nose and surrounding areas. Ø Comprehensive detailed 3D procedure planning with possibility to prepare and consider several scenarios quickly; Ø Follow-on illustration-piloted endoscopy Ø Real-time processing and fusion of the 3D MSCT data and endoscopic video that helps preventing undesirable intrusions into sensitive areas with instruments during surgical procedures Ø Additional visual tools about objects examinedimplemented in system, like layers, volume, surface and distances; Ø Tangible and realistic models produced with additive manufacturing technologies.
Experience gained from previous studies and from tool implementation, enables further development of specialized tools like algorithm for detecting abnormal patterns and comparative studies considering classic rhinologic procedures.
Acknowledgement The work presented in this paper was financially supported by the Ministry of Science, Education and Sports of the Republic of Croatia through the scientific research projectsNo. 152-1521473-1474 (Advanced Technologies of Direct Manufacturing of Polymeric Products) and No. 108-1521473-0339(Customized Implants) as a parts of the same research programme framework No. 1521473 (Rapid Production – From Vision to Reality).
References 1. KLAPAN I, ŠIMIČIĆ LJ, RIŠAVI R, BEŠENSKI N, BUMBER Ž, STIGLMAJER N, JANJANIN S, Orbit 20 (2001)35. – 2. RIŠAVI R, KLAPAN I, HANDŽIĆ-ĆUK J, BARČAN T, Int J Pediatr Otorhinolaryngol, 43 (1998) 271. – 3. KLAPAN I, ŠIMIČIĆ LJ, BEŠENSKI N, BUMBER Ž, JANJANIN S, SRUK V, MIHAJLOVIĆ Ž, RIŠAVI R, MLADINA R, Am J Otolaryngol, 23 (2002) 27. DOI: [10.1053/ajot.2002.28768]. – 4.KLAPAN I, VRANJEŠ Ž, RIŠAVI R, ŠIMIČIĆ LJ, PRGOMET D, GLUŠAC B, Ear Nose Throat J, 85 (2006) 318. – 5. RAOS P, GALETA T, Rapid Prototyping. In: KLAPAN I, KOVAČ M (Eds) 2nd Croatian congress ontelemedicine with international participation –TeleMED (Fakultet elektrotehnike i računarstva, Zagreb, 2004). – 6. RAOS P, GRIZELJ B, CUMIN J, J ManufE, 140 (2008) 40. – 7. ELOLF E, TATAGIBA M, SAMII M, ComputAided Surg, 3 (1998) 89. DOI: [10.3109/10929089809148134]. – 8. GEBHARDT A, Understanding Additive Manufacturing (Hanser Publishers, Munich, 2012). – 9. PETZOLD R, ZEILHOFER H-F, KALENDER WA, Comput Med Imaging Graphics, 23 (1999) 277. – 10. BAGARIA V, RASALKAR D, BAGARIA SJ, ILYAS J, Medical application of rapid prototyping - A new horizon. In: HOQUE ME (Ed) Advanced application of rapid prototyping technology in modern engineering (Intech, Rijeka, 2011).DOI: [10.5772/20058]. – 11. PILIPOVIĆ A, RAOS P, ŠERCER M, Int J Adv Manuf Tech, 40 (2009) 105. DOI: [10.1007/s00170-007-1310-7].– 12. PILIPOVIĆ A, RAOS P, ŠERCER M, Teh Vjesn – Tech Gaz, 18 (2011) 253. – 13. GALETA T, KLJAJIN M, KARAKAŠIĆ M, Stroj Vestn – J Mech E, 10 (2008) 725. – 14. KLAPAN I, ŠIMIČIĆ LJ, RIŠAVI R, PASARI K, SRUK V, SCHWARZ D, BARIŠIĆ J, J Telemed Telecare, 8 (2002) 125. – 15. KLAPAN I, ŠIMIČIĆ LJ, RIŠAVI R, BEŠENSKI N, PASARIĆ K, GORTAN D, JANJANIN S, PAVIĆ D, VRANJEŠ Ž, Otolaryngol Head Neck Surg, 127 (2002) 549. – 16. KLAPAN I, RAOS P, GALETA T, ŠIMIČIĆ LJ, LUKINOVIĆ J, VRANJEŠ Ž, MALEŠ J, BELINA S, ĆUK V, Application of advaced virtual reality and 3D computer assisted technologies in computer assisted surgery and tele-3D-computer assisted surgery in Rhinology. In: KIM J-J, (Ed) Virtual Reality (Intech, Rijeka, 2011).DOI: [10.5772/12989]. – 17. NN, 3D-DOCTOR Medical imaging software, accesed 1.06.2012. Available from: URL: http://www.ablesw.com/3d-doctor/– 18. KLAPAN I, VRANJEŠ Ž, PRGOMET D, LUKINOVIĆ J, Coll Antropol, 32 (2008) 217. – 19. KNEZOVIĆ J, KOVAČ M, KLAPAN I, MLINARIĆ H, VRANJEŠ Ž, LUKINOVIĆ J, RAKIĆ M, Coll Antropol, 31 (2007) 315.
P Raos Mechanical Engineering Faculty in Slavonski Brod, University J.J. Strossmayer of Osijek, I.B. Mažuranić 2, HR- 35000 Slavonski Brod e-mail: praos@sfsb.hr
ADITIVNA PROIZVODNJA MEDICINSKIH MODELA – PRIMJENA U RINOLOGIJI
SAŽETAK U radu se daju preporuke i smjernice za primjenu softvera za obradu medicinskih slikovnih zapisa u dijagnostici patologije glave i vrata, kao i postupka izrade fizičkih medicinskih modela tehnikama aditivne proizvodnje (brze izradbe prototipova), imajući u vidu unapređenje kirurškog zahvata, maksimalne sigurnosti njegove provedbe, te bržeg postoperativnog oporavka bolesnika. Ovaj pristup je potvrđen u dva obrađena slučaja bolesnika. Pritom su u liječenju korišteni i inteligentni algoritmi za prepoznavanje patologije s pomoću računalnog sustava za virtualnu trodimenzijsku i endoskopsku potporu u rinologiji s odgovarajućom vizualizacijom anatomije i patologije unutar područja nosa, paranazalnih sinusa i baze lubanje.